The route to Net Zero is expected to increase demand for electrical steels for both motors and transformers using high grade ferro-silicon. Downwards pressure on emissions from standard steel production will also support higher quality ferrosilicon demand. CRU Consulting has experience in helping ferrosilicon and other ferroalloys producers or consumers understand the medium and long-term future supply, demand, pricing and cost competitiveness environment globally and regionally. CRU Consulting has applied its expertise in assessing emissions and ESG policy impact in this sector. Contact us to discuss your challenges and how we can help you.
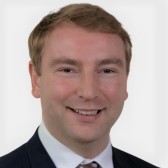
Author Aurelien Henry
Senior Consultant View profile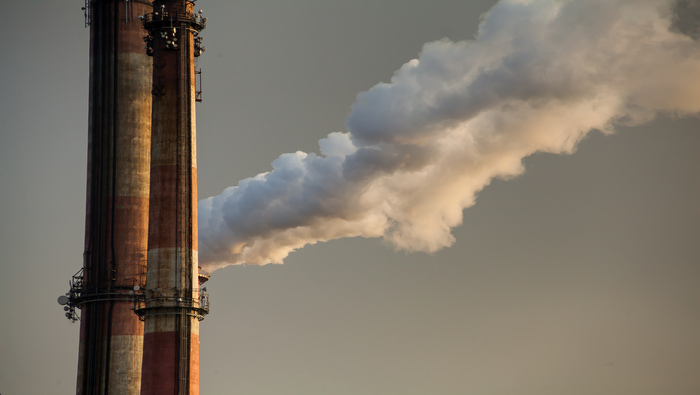
ESG-driven changes in steel will impact ferrosilicon demand
Ferrosilicon (FeSi) is an alloying element in the production of steel, and iron castings. Today, standard grade ferrosilicon (FeSi 75%) is the most common product by volume globally. Premium grade ferrosilicon (e.g. high purity or low aluminium ferrosilicon) are produced in much lower volumes and used in speciality grade steels, such as electrical steels.
Steel production has been under increasing scrutiny and pressure for its carbon footprint, as it is widely agreed the industry represents around 7% of global greenhouse (GHG) emissions. The main steel-consuming sectors, automotive and construction, are key activities that will need to demonstrate the route to Net Zero by adopting higherperformance, low-carbon steels thereby reducing sector-wide emissions and optimising steel intensities in end-uses. One of the consequences is that there will be an increasing emphasis on the quality of ferrosilicon alloys used and efficiency gains they can bring as part of more comprehensive decarbonisation strategies in both sectors.
The future ‘green economy’ is also likely to benefit electrical steel, also called silicon steel, with increased demand for high-grade electrical steel to come from the EV and renewable energy sectors. EVs use approximately double the weight of electrical steel compared to conventional ICE vehicles as it is needed in motors. Electrical steel is also needed in transformers that will be in-demand to expand EV-related infrastructure such as charging stations and support more widely the growth of the renewable energy sector, which has a higher electrical steel intensity than fossil fuel-based energy.
China is the by far the main producer and consumer of ferrosilicon worldwide. It is largely self-sufficient and a net exporter of ferrosilicon. Regions like Europe (defined here as EU27 and EFTA countries) and the US are however net importers. As shown in Figure 1, ferrosilicon imports for Europe and the US in 2022 represented 40% and 65% of apparent consumption respectively. Note that European producers focus, wherever possible on premium grade ferrosilicon to get higher value product mix, and limit, to some degree, competition with volume producers of standard grade.
Decarbonisation efforts will ultimately reach all the steel value chain
Until recently, efforts by governments to address climate change have primarily focused on reducing GHG emissions produced within their own borders. Emissions Intensive Trade Exposed industries (EITEs) like steel and ferroalloys production are impacted by carbon costs within the perimeter of, for example, the European Emissions Trading System (EU ETS) or the regional Western Climate Initiative (linking California and Québec ETS systems). This approach fails to address the emissions associated with imported goods and services. Therefore, governments are now developing policies to address this “carbon loophole” by accounting for the carbon intensity embedded in internationally traded products (particularly industrial products from energy-intensive industries). These new policies include the European Union’s Carbon Border Adjustment Mechanism (CBAM), the US-EU Global Arrangement on Sustainable Steel and Aluminium (GASSA), and the United Nation’s Industrial Deep Decarbonization Initiative (IDDI).
Initially targeting Scope 1 emissions, these polices will need to subsequently extend the carbon cost burden to Scope 2 and even Scope 3 emissions to stimulate decarbonisation efforts all along supply chains. This will ultimately put a greater emphasis on the carbon footprint from increasingly in-demand, emissions-intensive products like ferrosilicon.
Ferrosilicon emissions remain regionally variable and will create new challenges for consumers and trade
Ferrosilicon production requires carbon to reduce the quartz and producers will use different reductant mixes among which coal, petroleum coke, semi-coke, or biomass (i.e. charcoal or woodchips). As a result, ferrosilicon producers have a wide range of Scope 1+2 emissions intensity, with differences in Scope 2 intensities driven by local grid configuration. Figure 3 shows country average Scope 1 and 2 emissions excluding bio-CO2 emissions range from below 1.0 tCO2/t FeSi to more than 10.0 tCO2/t FeSi (even greater variability is observed at producer-level). Scope 1 emissions intensity are broadly similar except for Brazilian production which relies mostly on charcoal in their process, significantly lowering their footprint. Hydro-based production that can be found in China or Scandinavia for example also displays a lower footprint than producers sourcing their electricity from a grid with a high share of gas- and coal-based power generation.
Importantly, patterns for raw materials use and power sourcing are evolving in the ferrosilicon sector. Some producers are increasing the use of biomass, carbon capture technologies are being considered and some producers have installed energy recovery systems to reduced electricity consumption. CRU is tracking these trends aimed at reducing Scope 1 and 2 for ferrosilicon producers.
For any questions and/or discussion, please reach out to Aurélien Henry, Associate Senior Consultant, Jorn de Linde, Senior VP, Steel or Clare Hanna, Senior Analyst Manganese Ore and Ferroalloys.
Explore this topic with CRU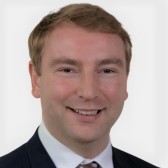
Author Aurelien Henry
Senior Consultant View profile